Altitude Training
Introduction
Since the 1968 Mexico City Olympics held at 2300m, altitude training has become an integral part of an elite athletes arsenal aiming to achieve peak performance (Daniels and Oldridge, 197012; Dill and Adams, 197114; Tonnessen et al., 201474; Solli, Tønnessen and Sandbakk, 201770; Mujika, Sharma and Stellingwerff, 201950). Although the validity of altitude training for elite athletes has continued to be debated (Lundby and Robach, 201644; Millet et al., 201948), the proposed adaptations cannot be underestimated (Ploszczyca, Langfort and Czuba, 201853; Sinex and Chapman, 201569). Balancing the acute and chronic physiological responses to altitude is essential for achieving the greatest benefit to performance (Sinex and Chapman, 201569). The levels of altitude are defined in Table 1, with the majority of the research focusing on moderate to high altitudes (Bonetti and Hopkins, 20093; Mujika, Sharma and Stellingwerff, 201950). To rationalise and interpret the physiological basis for altitude training it is important to understand that as altitude rises, both the barometric pressure (Pb) and partial pressure of oxygen (PiO2) decreases which leads to a reduction in the availability of oxygen that can be delivered to skeletal muscle, despite the concentration of oxygen remaining constant (20.9%) inducing hypoxic conditions (Sinex and Chapman, 201569; Kenney, Wilmore and Costill, 201939).
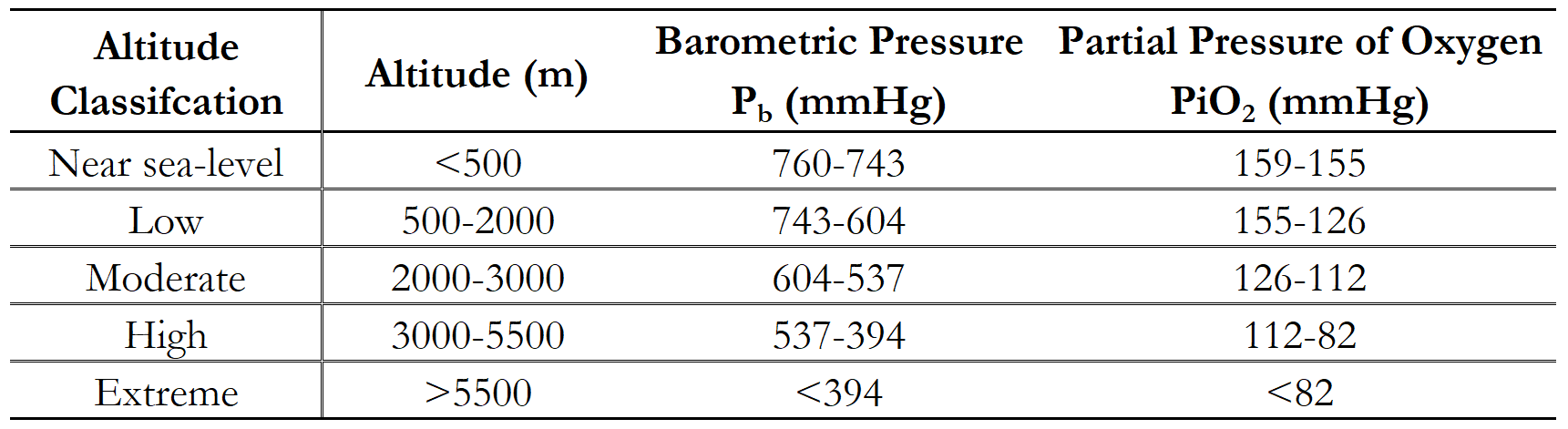
Table 1: Classification of altitude adapted from Kenney, Wilmore and Costill (2019)39 and Sinex and Chapman (2015)69.
These changes result in several haematological (Levine and Stray-Gundersen, 199240; Ploszczyca, Langfort and Czuba, 201853), cardiovascular (Naeije, 201051; Shah and Coplan, 201968) and ventilatory (Townsend et al., 201675) adaptations along with other benefits including increased buffering capacity (Gore, Clark and Saunders, 200725; Mizuno et al., 199049; Gore et al., 200126), oxidative enzyme activity (Terrados et al., 199073; Katayama et al., 200437), increased capillary density (Mizuno et al., 199049; Vogt et al., 200177), myoglobin concentration (Terrados et al., 199073; Zoll et al., 200681) and mitochondrial capacity (Desplanches et al., 199313; Geiser et al., 20023) which can occur both acutely and chronically.
These adaptations are important as they may offer an advantage above and beyond those which occur at sea-level (Bonetti and Hopkins, 20093; Mujika, Sharma and Stellingwerff, 201950). However, these adaptations are dependent on several factors such as the level of altitude, time spent at the given altitude, training intensity, training load, fatigue, iron status (serum ferritin >50 µg.L-1 (Clénin et al., 201511)), iron supplementation (50-100 mg/day (Garvican-Lewis et al., 201620)), medical history and individual responses (Friedmann et al., 200519; Wilber, Stray-Gundersen and Levine, 200780; Mazzeo, 200847; Friedmann-Bette, 200818; Chapman, 20137; Schmitt et al., 201866; Garvican-Lewis et al., 201822; Brocherie, Schmitt and Millet, 20174).
Christof Sonderegger von Photoplus.ch, CC BY-SA 3.0, via Wikimedia Commons
Physiological Adaptations to Altitude
Hypoxia-induced Factor-1α (HIF-1α)
A comprehensive over view of the physiological responses in altitude are outlined in Table 2, however despite the various adaptations that contribute to improved performance due to altitude training, the predominant adaptation which athletes and coaches strive for are the haematological changes that occur with decreasing PiO2 …
Responses